Controlling “chemotaxis” in environmental bacteria to
facilitate agricultural and environmental improvement
The Metabolic Engineering Laboratory led by Professor Junichi Kato specializes in cutting-edge biotechnology. Its precursor can be traced to the Department of Brewing of Hiroshima High Institute of Technology launched in the pre-World War II period. According to Prof. Kato, “The Department was working to discover microbes that could produce large amounts of alcohol, clarify their biofunctions and then culture the microbes to make effective use of them.” In the same direction, the Kato Laboratory is committed to education and research aiming to discover and elucidate excellent biofunctions of microbes, and then culture these microbes to make the most of them for the benefit of humanity.
In particular, Prof. Kato primarily focuses on “research aimed at establishing sustainable agriculture, through the effective use of biofunctions possessed by environmental bacteria.” This work is characterized by an approach of “understanding how microbes in soil perceive plants.” In the realm of nature, living things are connected in some way to other life forms through biological interactions—such as, the prey-predator relationship, parasitism, competition, and infection in the case of bacteria. Accordingly, if we can understand “how microbes perceive plants” as mentioned above, we can prevent plants from becoming infected with phytopathogens. We can also use good bacteria to promote the growth of a plant even with a smaller amount of fertilizer, by inducing these bacteria to gather to the plant. Establishing such methods may enable us to emerge from the current intensive agriculture that is dependent on chemical fertilizers, paving the way for the establishment of sustainable agriculture that will be less independent of fertilizers and agricultural chemicals. Prof. Kato has set it as his research goal to embody this idea.
For this reason, he pays attention to “chemotaxis,” a biological function of microbes. Chemotaxis refer to the purposeful behavioral responses of environmental bacteria. They can gather toward “what they like (attractants),” while avoiding from “what they don’t like (repellents).” Research into chemotaxis has a very long history, dating back to the early 18th century. Antony van Leeuwenhoek, a Dutch scientist who invented the microscope, found that microbes exhibited chemotaxis. However, it was not until the 21st century that this finding began to be experimentally demonstrated, according to Prof. Kato. He explains the reasons why it took so many years, saying, “One reason was speed. Microbes swim at a speed of 0.1 mm per second. Given such low motility, scientists wondered what chemotaxis would be good for. However, later it was experimentally demonstrated that the absence of chemotaxis caused a delay in biological interactions. Furthermore, he offers supplementary reasons, citing indirect evidence including the following: environmental bacteria require more than 50 genes to exhibit chemotaxis; so far, microbes have been under natural selection pressure for more than three billion years; and a majority of environmental bacteria existing today exhibit chemotaxis. Consequently, the necessity and importance of chemotaxis is now obvious.
Meanwhile, chemotaxis is expected to be beneficial not only for agriculture but also for environmental cleanup. Prof. Kato says, “When you introduce a microbe capable of degrading environmental pollutants, if the microbe could discover and degrade the pollutants by itself, wouldn’t it be wonderful? Imagine if once you sprayed such a microbe on farmland, the microbe could detect and degrade the environmental pollutants on its own. It might sound a bit like a manga [cartoon] storyline. I’m rather fond of the manga genre, and if we could create a microbe of this kind, it would be surprising for everyone, which motivates us to pursue this research.”
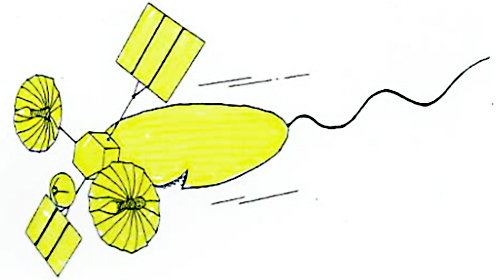
If a microbe could detect environmental pollutants by itself…
Discovering many facts by studying the relationship between plant infection with Ralstonia solanacearum and chemotaxis
As a subject of research into chemotaxis, Prof. Kato handles Ralstonia solanacearum, a bacterium that poses the greatest threat among plant pathogens. If crop species, such as tomato, potato, eggplant, tobacco or banana, are infected with this bacterium, it causes wilt disease in these plants, causing them to wilt and eventually die. Prof. Kato says that Ralstonia solanacearum has damaged plants around the world. A common countermeasure is to carry out soil disinfection. However, with the banning of the use of methyl bromide, an effective disinfectant, the establishment of a new technology to control bacterial wilt disease is required. Given this situation, Prof. Kato’s research group has come to consider the feasibility of utilizing chemotaxis to address this issue.
“It is thought that Ralstonia solanacearum lurks in the soil for an extremely long period of time, and if a plant is planted in that soil, the bacterium will move toward the plant and infect its root, thus causing bacterial wilt disease. So what kind of substances released from the plant can Ralstonia solanacearum detect, causing it to migrate toward the host plant? We thought that if we could discover these substances, this methodology might allow us to develop technology to defend plants from infection.”
Specifically, Prof. Kato’s research group worked on clarifying the attractants secreted from plants. As a result of the investigation, they found that amino acids and organic acids often attracted Ralstonia solanacearum (as shown in Fig. 3 below).
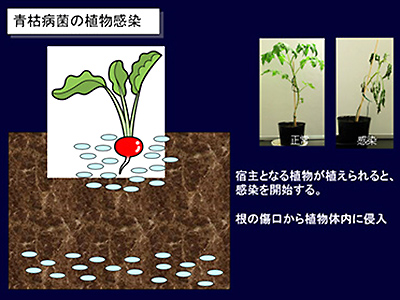
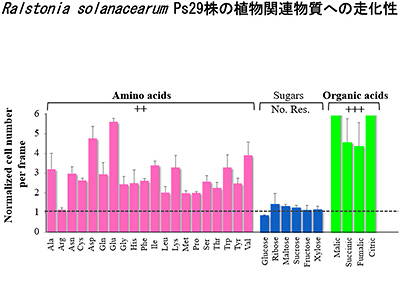
The Y-axis indicates chemotactic strength. The value of 1 or more indicates an attractant response, while less than 1 indicates a repellent response.
Here, let us introduce the mechanism whereby bacteria display chemotaxis. A simplified conceptual rendering is shown in Fig. 4 below. According to Prof. Kato, “chemotaxis sensors” exist in membranes surrounding microbe cells. When an attractant is detected by the sensor, it sends a signal to the cell-cell communication system indicating the presence of the attractant. Then, the information is processed to control the rotational direction of the flagellar motor. As a result, bacteria will gather toward attractants, or conversely, escape away from repellents.
He also points out that a single bacterium has several sensors. “If only we could identify what substances each sensor detects, we would be able to understand the individuality of the bacterium.” In line with this idea, his research group conducted detailed investigation of the sensors of Ralstonia solanacearum.
It is known that Ralstonia solanacearum has 22 chemotactic sensors. However, when the research was launched, initially Prof. Kato’s group only understood what was sensed by two of the 22 sensors. To pursue in-depth studies, the group adopted a method of breaking these 22 sensors through genome editing. Prof. Kato explains: “We created 22 sets of samples in which one of the 22 sensors was broken. In addition, we made another 22 sets of samples in stages, each of which had between one and twenty-two defective sensors. By using these two sets, we examined how the chemotaxis changed.” As a result, his group succeeded in independently clarifying what each of the individual sensors detected.
Next, Prof. Kato’s group carried out experiments to understand what was involved in the plant infection. “For instance, if you find that plant infection is reduced when a mutant strain with no amino acid sensor is introduced, you can conclude that chemotaxis to amino acids is involved in the infection,” says Prof. Kato. Based on this idea, his research group proceeded with study into the relationship between the sensors and plant infection, using many kinds of mutants (as shown in Figs. 5 and 6 below).
As a consequence, his group succeeded for the first time in the world in experimentally demonstrating that Ralstonia solanacearum detects malic acid secreted from plants and migrates toward them, causing plant infection.
Furthermore, Prof. Kato’s group conducted application experiments, through which they discovered that applying about 1 mM of malic acid to cropland would inhibit plant infection on the land. He says “Almost all plants secrete malic acid, which is used as a food additive that has proved safe and yet inexpensive. To put it another way, we have begun to learn that we may control Ralstonia solanacearum without depending on agricultural chemicals, by applying this safe and inexpensive food additive to farmland.” This discovery also marked the first step toward the realization of sustainable agriculture, which he aims to achieve.
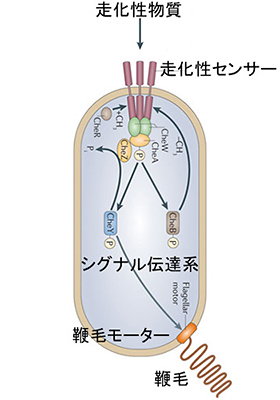
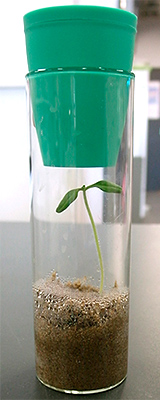
Tomato seedlings are cultivated in sterilized sandy soil, and R. solanacearum is planted in a spot 3 cm away from the seedlings. Subsequently the number of seedlings that wither due to bacterial wilt disease is counted to evaluate the infectious ability.
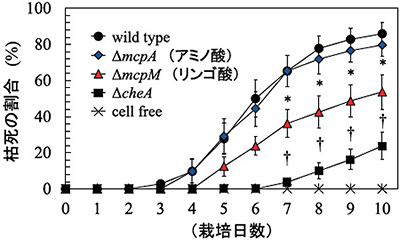
By using the method described in Fig. 5, we evaluated the ability to infect tomato seedlings of the MAFF106611 strain, its mutant strain having chemotaxis to amino acid (ΔmcpA), its mutant strain having chemotaxis to L-malic acid (ΔmcpM), and a general chemotaxis mutant strain (ΔcheA). The Y-axis indicates the rate of tomato seedlings that withered and died. The X-axis indicates the number of days of cultivation.
Research that is instrumental in achieving the SDGs—
succeeding in producing bacteria that facilitate environmental purification
Here is yet another achievement. Among the initial plans of Prof. Kato was the construction of “super pollutant degraders with a radar function,” depicted in Fig. 1 in the above section. Environmental purification by harnessing biological functions is called “bioremediation.” Prof. Kato hit upon the idea that chemotaxis may be effectively applied to this field, and he later succeeded in producing such bacteria akin to something out of a manga (cartoon) story.
He says “In conducting this research, I decided to use an environmental pollutant called trichloroethylene (TCE). Since TCE has a property of dissolving oils, it is often used in vehicle manufacturing plants, dry-cleaning plants, etc. TCE is also hard to corrode. This means that this pollutant is not easily degraded by microbes, and thus continues to accumulate in the soil. Moreover, if TCE enters an organism, it will change into a carcinogenic substance. In Western countries, where groundwater is frequently used as a source of drinking water, contamination with trichloroethylene has become a matter of concern for around the last 30 years.”
To begin with, Prof. Kato’s group undertook “screening” to discover microbes that could degrade TCE. Prof. Kato remembers that screening equipment unique to Hiroshima University proved very effective at that time. Subsequently, the group eventually found microbes that degraded trichloroethylene.
“If this microbe capable of degrading this environmental pollutant accumulates toward TCE, we would be able to clean up the environment in a more effective manner. With this idea in mind, we studied chemotaxis to trichloroethylene, but only to learn that the microbe was migrating away from the pollutant. It was not an attractant but a repellent. Disappointed with the result, we were just about ready to give up.”
Nevertheless, we subsequently discovered a sensor that was necessary for migrating away. We then conducted an experiment using a mutant strain that did not possess the sensor, and obtained an unexpected result. Prof. Kato says, “We found that this bacterium not only had a sensor for migrating away from TCE, but also a sensor for accumulating toward TCE. We wondered why the bacterium moved toward trichloroethylene, which is a toxic substance. To examine the reason, we conducted further experiments using mutant strains, in order to discover a gene with a sensor for accumulation.”
This achievement paved the way for the construction of “super pollutant degraders with a radar function.” He explains that “If the sensor for accumulation is strengthened, the bacteria will gather toward high concentrations of trichloroethylene. By incorporating this chemotaxis sensor gene into a bacterium that degrades environmental pollutants, we can create a microbe capable of detecting and degrading trichloroethylene.” Kato Laboratory has now constructed “super pollutant degraders with a radar function” by introducing chemotaxis sensor genes for trichloroethylene and chloroaniline, respectively, into P. putida F1 (a strain with trichloroethylene degradation activity), and P. putida T57 (a strain with chloroaniline degradation activity). Prof. Kato expects that, through this chemotaxis research, his laboratory can contribute to achieving Goals 12, 13 and 15 of the 17 Sustainable Development Goals (SDGs).
As mentioned so far, Prof Kato has accomplished a variety of research outcomes. His research activities are motivated by his hope to “give people surprise” and “serendipity [unexpected findings] that he encounters from time to time.”
He says with a smile, “I discovered the fact that microbes are strongly attracted to boric acid, for the first time in roughly 300 years of research history. The catalyst for this discovery was boric acid glass, which we usually used for experiments. In this manner, this research provides us with opportunities to make unexpected discoveries. This is one of the pleasures of the research."

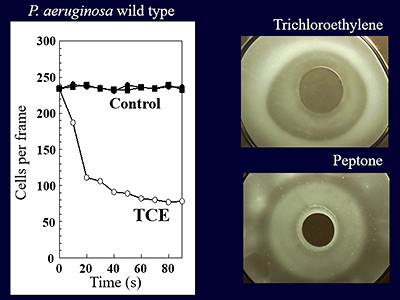
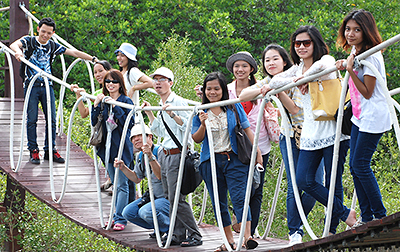
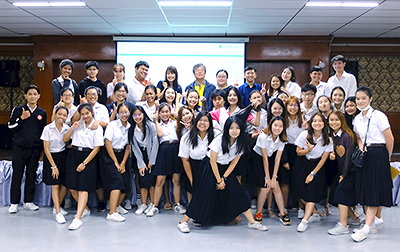
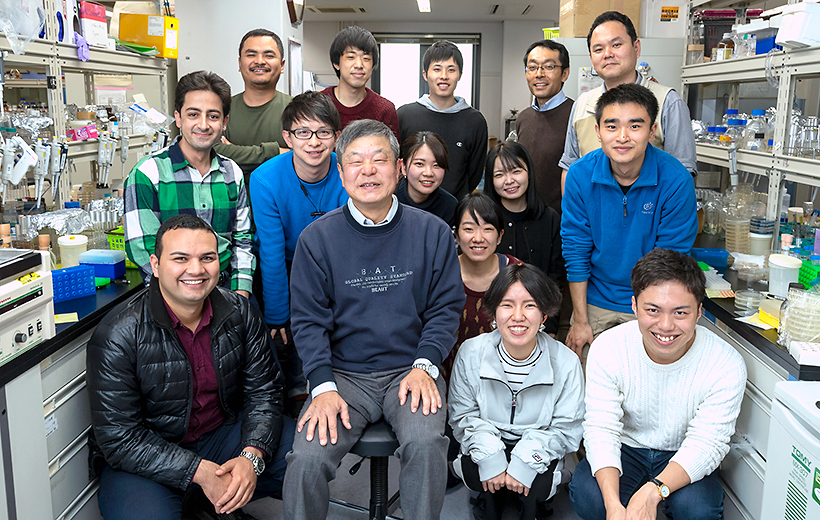